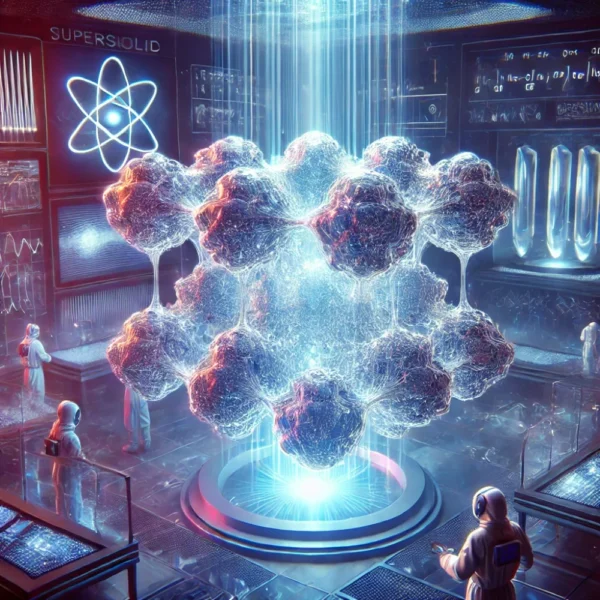
Discover how researchers have transformed light into a supersolid using polariton condensates in photonic crystal waveguides. This breakthrough features technical milestones like a density modulation precision of a few parts per thousand, threshold injection rates around 50 ps⁻¹, and a coupling strength of ~1 meV—paving the way for revolutionary advances in quantum computing, telecommunications, and advanced materials.
In a breakthrough that seems more of science fiction than fact, researchers have successfully transformed light into a supersolid—a state of matter that marries the crystalline order of a solid with the frictionless flow of a superfluid. Traditionally, supersolids were observed only in ultracold atomic gases at temperatures near absolute zero. By harnessing the properties of polariton condensates in photonic crystal waveguides, scientists have achieved this exotic state using light, setting the stage for revolutionary advancements in quantum technologies.
The Dual Nature of Supersolids
Supersolids are fascinating because they defy everyday understanding. In a typical solid, atoms are locked in a rigid lattice, while in a superfluid, particles flow without resistance. A supersolid, however, exhibits both properties simultaneously—it maintains a periodic, crystalline structure while allowing for frictionless, coherent flow. This duality implies that, despite the material’s ordered arrangement, it can dynamically transport energy and information without the usual limitations of viscosity.
Polariton Condensates: The Secret Ingredient
At the heart of this innovation are polaritons—quasiparticles that emerge when light (photons) couples strongly with excitons (bound electron–hole pairs) inside a semiconductor like aluminum gallium arsenide. When a laser irradiates the semiconductor embedded with photonic crystal waveguides, polaritons are created and can condense into a coherent quantum state much like a Bose–Einstein condensate, but at more accessible conditions.
The photonic crystal waveguides, with their meticulously engineered ridge patterns and periodic lattice structure (with characteristic periodicities on the order of a few micrometers), confine the polaritons and force them into a spatially modulated arrangement. This induced density modulation is the signature of supersolidity—the system spontaneously breaks continuous translational symmetry while maintaining long-range coherence. Researchers have measured this modulation with an impressive precision of a few parts per thousand.
Technical Aspects
Injection Rates & Thresholds:
Experimental setups indicate that the polariton condensation threshold is marked by an injection rate around 1 ps⁻¹. However, when the injection rate reaches approximately 50 ps⁻¹, a second emission threshold appears. This additional threshold signals the onset of nonlinear polariton scattering into finite momentum states—a clear hallmark of the transition into a supersolid state.
Coupling Strength:
In these systems, the interaction between the confined photonic modes and the excitonic resonances is characterized by a coupling matrix element on the order of ℏU₀,₁ ≈ 1 meV. This parameter is crucial as it governs the strength of the light–matter interaction and influences the stability of the polariton condensate.
Density Modulation Precision:
Using advanced spectroscopic techniques, researchers quantified the density modulation of the polariton condensate, demonstrating spatial order with a precision of a few parts in a thousand. This precise measurement confirms the breaking of translational symmetry—a defining trait of the supersolid phase.
Implications for Quantum Technologies
The successful transformation of light into a supersolid opens up a host of exciting opportunities:
Quantum Computing:
The global phase coherence combined with a crystalline structure could inspire new architectures for quantum computing, where robust qubits benefit from both ordered stability and frictionless flow.
Telecommunications and Sensors:
The controlled manipulation of light in these engineered structures could lead to devices with enhanced signal integrity and lower energy loss, critical for next-generation optical communications and high-performance sensors.
Advanced Materials:
The novel state of matter offers the potential for developing materials that leverage the dual properties of order and superfluidity—leading to innovations in energy-efficient devices, precision machinery, and even novel cooling systems.
A Bright, Solid Future
In summary, this research confirms that light can indeed be transformed into a supersolid through the careful engineering of polariton condensates in photonic crystal waveguides. By combining traditional photonic techniques with modern quantum insights, researchers have not only deepened our understanding of exotic states of matter but also opened new pathways toward practical quantum technologies. As scientists continue to refine these methods and explore the rich dynamics of driven-dissipative systems, the future of quantum materials looks both bright and remarkably solid.